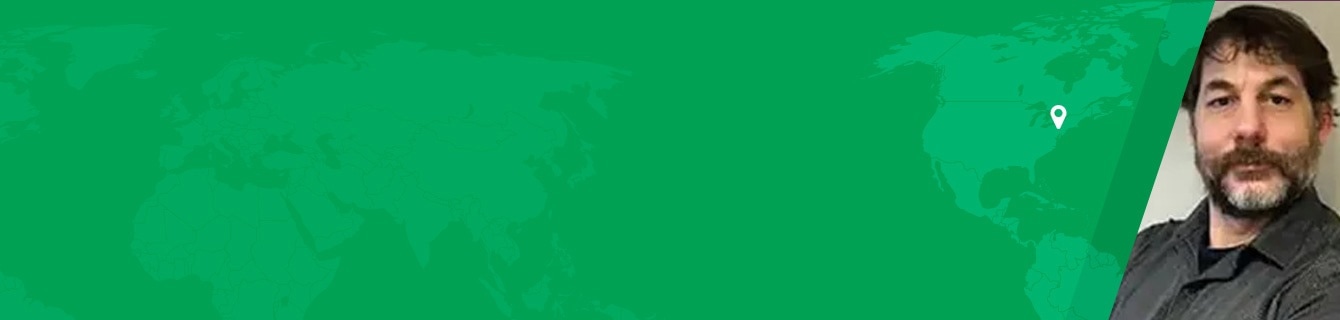
mRNA vaccines are a cornerstone of modern healthcare – and this has perhaps never been more true than in the last two years. An essential methodology supporting the fight against the COVID-19 pandemic, mRNA vaccines also have applications far beyond tackling SARS-COV-2.
Brian Matlock, a senior product application specialist at Thermo Fisher Scientific, que es mejor diazepam o myolastan provides insight into the different mRNA vaccine methodologies used today. In this article, he explores the various research areas that are continuing to work towards more efficacious and tolerable mRNA vaccines to fight against emerging pathogens.
Brian details the four major steps involved in producing mRNA vaccines and describes how Thermo Scientific UV-Vis spectrophotometers are a vital and integral part of the vaccine development and manufacturing workflow.
Please could you introduce yourself and outline your role within Thermo Fisher Scientific?
My name is Brian Matlock, and I am a senior product application specialist at Thermo Fisher Scientific. I specialize in the development of UV-Vis technology for applications in pharmaceuticals, biosciences, academia, and industry.
During my 13 years at Thermo Fisher Scientific, I have been fortunate enough to work closely in biotechnology, molecular biology and PCR, among other areas, and prior to this, I spent eight years working as a scientist for various pharmaceutical, biotech and life sciences companies.
Image Credit:Shutterstock/DanRace
Could you provide a brief history of vaccine research?
Vaccine research began in the late 18th century with Edward Jenner’s work on the smallpox vaccine. It was followed up by Louis Pasteur’s work, which centered around the idea of isolating a pathogen, and, subsequently, inactivating that pathogen before injecting it back into organisms to provide protection against that pathogen. In its simplest form, vaccines present our bodies with inactivated pathogens – or parts of pathogens – to train our immune systems so that when it is presented with that pathogen again, our bodies will be able to fight off the infection.
In the 1950s, the foundations of the traditional vaccine platforms that we are familiar with today were formed. This was enabled by innovations in the propagation of viruses and cell culture. The traditional vaccine platforms are virus-based vaccines and protein-based vaccines.
What are virus-based vaccines?
Virus-based vaccines can be broken into a couple of categories. Inactivated virus is where the pathogenic virus is propagated in cell culture, isolated, and then inactivated with heat. An example of this type of vaccine is the polio vaccine which was produced on a large scale. Vaccination for polio begun in the 1950s.
The second category of virus-based vaccines is the use of live attending awaited viruses. The steps involved here are the isolation of the pathogenic virus and the passing of that virus multiple times through various cell lines. During this process, the virus starts to accumulate mutations and reduces its virulence. An example of this approach is the MMR vaccine and some of our seasonal flu vaccines.
Protein-based vaccines involve virus-like particles, individual proteins or protein sub-units. Nucleic acid therapies have been a very popular approach to creating new medicines since the nineties when advances in the genomics fields first enabled innovation in this area. At that time, the use of nucleic acids as vaccines was first investigated, and initially, all the work was done using DNA because it is much more stable than RNA.
Plasma vectors expressing the antigen of interest were introduced into cells, where they would then be transcribed and translated into the protein antigens. DNA delivery into cells within the body became a large barrier to overcome. When this is performed either in vivo or outside of the body, electroporation is used as an efficient way to introduce DNA into cells. However, this is not an efficient approach to use in in vivo delivery.
RNA also initially presented several challenges: most notably, its instability. Innovations over the last decade have allowed RNA to become a viable vaccine platform today. The final category is the use of replicating or non-replicating viral vectors. This platform evolves cloning antigen genes into well-characterized viral vectors like adenovirus.
Image Credit:Shtterstock/KaterynaKon
Can you tell us how you got to mRNA vaccines from the unstable RNA vaccines?
In the non-replicating mRNA vaccine methodology, the important components of the in vitro transcription construct or IVT consists of a five-prime cap, which aids an efficient translation of the transcript to protein. Subsequently, there will be a 5' untranslated region, followed by an open reading frame, which encodes the antigen. Finally, there will be an untranslated 3' region and a poly(A) tail. Once this IVT construct is delivered into the cell, it will produce the antigen to elicit an immune response until, finally, the mRNA degrades.
Another mRNA vaccine platform encodes viral machinery, which allows this IVT construct to replicate itself once it enters the cell. This allows for much less mRNA to be used for injection because intracellular amplification of the RNA takes place.
Important advances have occurred that have optimized IVT mRNA pharmacology. They include capping analogs, capping enzymes, regulatory elements that are added to the UTRs or untranslated regions, the length of the poly(A) tail, modified nucleosides and then optimization within the antigen.
What are some of the key research areas that brought these platforms to the forefront of producing some of the important COVID-19 vaccines that are in use today?
There are three primary areas of focus when we consider the steps that must happen once the mRNA is injected into our body to elicit an immune response. The first is to optimize the RNA sequence – thus that the rate of translation is high and the half-life within the cell is long.
The second is how the IVT mRNA is purified. It is important that it is free from any double-stranded DNA or transcription components. The IVT mRNA reaction requires several enzymatic steps, and purification happens post-reaction. HPLC and other methods are employed to purify the mRNA vaccines.
The final area of research that has allowed these platforms to become front and center of vaccine production for COVID-19 has been mRNA delivery into cells. Many areas have been exploited here, including the use of nanoparticle lipids, polymers, and peptides. These carrier molecules improve mRNA stability, they help reduce the toxicity, and facilitate mRNA translocation into the cytoplasm.
On-demand webinar: mRNA vaccine development and manufacturing workflows enabled by UV-Vis spectroscopy
Watch the On-Demand Webinar Here
What makes the mRNA vaccine platform so attractive to manufacturers and labs?
Within the modern mRNA vaccine production workflow, the mRNA platform has clear advantages, such as enabling short development times and featuring inexpensive manufacturing steps. These steps are also cell-free, which makes them safer, and sequence-independent, meaning the manufacturing steps remain consistent from vaccine to vaccine. Some of the traditional vaccine platforms will require changing the steps depending on the vaccine.
What is the production process of the mRNA vaccine workflow?
The first part of the production process is making that mRNA IVT construct. To do this, it is essential to first isolate viral nucleic acid or the pathogen of interest.
Typically, the genome is sequenced, and then the antigen is selected. This is the piece on the virus or pathogen that the body is going to detect. Finally, there are several cloning steps involved in making the expression IVT construct. Once this construct is made, the user can then move on to the actual IVT or in vitro transcription step of the process.
Image Credit: Thermo Fisher SCIENTIFIC
This entails first linearizing the template DNA and then setting up the IVT reaction. This reaction will consist of that template, linearized DNA, dNTPs, and then RNA polymerase. The reaction forms the mRNA construct.
After the construct is formed, purification becomes very important, which is the next stage. It is therefore critical to remove the IVT reaction components, double-stranded DNA, enzymes and partial transcripts. It has been demonstrated that many of these components will affect how the patient’s body responds to the vaccine and ultimately decrease its effectiveness.
In many cases, HPLC is used here to purify these constructs, but there are plenty of other strategies that people are exploring as we continue to try to scale up this process. The final step of vaccine production is the formulation, where any additional components are added. These additional components will either help further stimulate an immune response, help deliver that mRNA into the cytoplasm of cells, or they will be components that improve the storage and stability long term of the mRNA.
Could you provide a brief background about UV-Vis spectroscopy and why it is important to this process?
In many of the steps I have outlined above, UV-Vis measurement is used to check the concentration and the purity of nucleic acids. UV-Vis has been a central technique used for a long time in this process because the aromatic rings within the nucleotides absorb UV light.
The amount of light these aromatic rings absorbed is directly related to the concentration of the nucleic acid. Beer's Law can be used to determine this, with appropriate coefficients and factors.
Full UV-Vis spectra not only provide users with the concentration but also offers a clear idea of purity and what else is in the sample. A range of different contaminants can be present in the sample, alter the spectrum, especially if they absorb light at different regions than the mRNA.
Therefore, full-spectrum data is used along with chemometric algorithms to help identify these contaminants. This helps researchers troubleshoot where the contaminants are coming through if they know the identity.
Image Credit:Shutterstock/thodonal88
Where does the NanoDrop One Spectrophotometer fit into the first two steps of the vaccine production workflow?
The pathogen or virus is isolated, and the nucleic acid is extracted. Once that extraction is done, it is then essential to obtain an idea of the purity and the concentration, which is undertaken on the Thermo Scientific™ NanoDrop™ Spectrophotometer. Prior to sequencing that genome, the antigen target is then identified.
There are several cloning steps in which the concentration and the purity is needed to set up those cloning steps to create the IVT construct. Finally, when we look at the IVT reaction itself, a concentration is important to determine the amount of template going into that reaction.
Is there anywhere else UV-Vis can be used in the production pipeline?
In the later steps in the production process, it is important to consider cuvette-based UV-Vis spectrophotometers and how they fit into the workflow. There are several ways in which the constructs can be created and those constructs amplified for the IVT reaction. The first way is to use oligonucleotides and cloning. It is vital to evaluate the 260/280 ratio because that allows for the determination of proteins that are in the sample, most notably, some of the enzymes used in the cloning process. This helps improve mRNA production and allows for faster vaccine production.
The Thermo Scientific Evolution™ One/One Plus and Evolution Pro Spectrophotometers are available with software and tools to enable easy compliance with regulations, including 21 CFR Part 11 requirements. These, therefore, fit into the later steps of vaccine production. The second way to amplify many of the components used in the IVT reaction is to use bacteria as a tool.
A) Absorbance B) Light scattering
Image Credit: Thermo Fisher SCIENTIFIC
Optical density measurements have been used with spectrophotometers for a long time, and this partnering allows us to monitor bacterial cell growth. The phases of cell growth are important to think about: the lag phase of cell growth is when the bacteria are adjusting to the media; the log phase is when the bacteria are healthy and are reproducing exponentially; and finally, the stationary is where their growth rate slows down. Finally, there is a death phase.
The use of optical density measurements allows us to determine the optimal time to induce expression in the cell and then to determine when to harvest the cell. Spectrophotometers are used here for reliable OD600 measurements, which allow us to calculate the number of bacteria in the culture.
GENESYS 50 Spectrophotometer
Image Credit: Thermo Fisher SCIENTIFIC
This is where the Thermo Scientific GENESYS™ Spectrophotometers excel. The GENESYS instruments are used to monitor cell growth and determine when the best time is to harvest the cells of interest. This also improves the efficiency of the vaccine production process, ultimately leading to a faster production cycle.
Any last comments?
In conclusion, though it may seem obvious to state, vaccines have been an instrumental tool for improving health in both human and animal populations. New vaccine platforms, like mRNA, show promise for shorter development times and potentially easier manufacturing steps. Crucially, they have allowed us to react quickly to emerging pathogens, which has been critical – particularly in the fight against COVID-19. UV-Vis Spectrophotometers, therefore, play an important role in both vaccine development and manufacturing.
About Thermo Fisher Scientific – Materials & Structural Analysis
Thermo Fisher Materials and Structural Analysis products give you outstanding capabilities in materials science research and development. Driving innovation and productivity, their portfolio of scientific instruments enable the design, characterization and lab-to-production scale of materials used throughout industry.
Sponsored Content Policy: News-Medical.net publishes articles and related content that may be derived from sources where we have existing commercial relationships, provided such content adds value to the core editorial ethos of News-Medical.Net which is to educate and inform site visitors interested in medical research, science, medical devices and treatments.